|
|
|
|
|
|
|
|
|
|
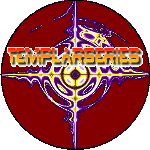 |
AGE-OLD STORY
|
 |
Figure 1: As we age, our skin
becomes more disorganised and inelastic, fat builds up in particular areas
and hair greys and recedes |
Ageing seems to be an invariable law of nature-it is something everyone
must come to terms with. But at least
scientists are beginning to explain the changes that
occur, and are even suggesting ways to turn back the clock
James Kingsland
WHY do we have to die? We are not like machines- our bodies
constantly repair and renew themselves. And unlike a brand new car,which
starts to wear out the moment it leaves the showroom, a newborn baby's immunity,
coordination, heart and circulation will actually "improve" during its first
decade. True, the chances of dying-mortality-are high around birth, but they
steadily decline until around 10 years of age. Mortality only starts to increase
again at puberty, when ageing begins in earnest. From then on it's a gentle
but slippery slope all the way to the grave.
Down the ages, countless sages and magicians have discovered "elixirs of
life", but none has survived to pass on the recipe. There may be some consolation
in knowing that one of the cell lines in our bodies is potentially immortal,
namely germ cells -sperm or eggs. These cells, and the DNA they carry
trace their ancestry right back in an unbroken line to the first organisms
that swarmed over the Earth more than 4 billion years ago. The other cells
in our bodies, the somatic cells, are merely the disposable slaves
of this immortal DNA. Only in recent years has medical science found a way
for somatic cells to achieve immortality, but the price is high. Henrietta
Lacks, a young woman from Baltimore in the US who died in 1951,lives on in
cell cultures around the world. She was terminally ill when some of her cancerous
cells were removed and, long after her death,they're still going strong.
Normally, it is very difficult to culture human cells for long periods, so
HeLa cells have made a huge contribution to our understanding of cell
biology.
 |
Figure 2: Free radicals (blue
spheres) damage DNA proteins and lipids all over the cell |
The immortality of germ cells and some cancerous cells suggests that ageing
is not inevitable. For us mere mortals, however the story is rather different.
And though the annual mortality figures seem to show that we are successfully
tackling ageing, the truth is that falling mortality in the industrial world
is largely due to improved health care and infant survival. While average
life expectancy in Britain has increased over the past century from 49
to 74 years for men and from 52 to 79 years for women, maximum life span
has improved only modestly. When Jeanne Louise Calment died in 1997, she
was the first human to have lived to a verified age of 122. But at the turn
of the 20th century, Thomas Emley Young, a former president of the Institute
of Actuaries in Britain, verified the case of a person who had lived to 113
years of age. So while we have boosted average life span substantially, with
the help of antibiotics and improved hygiene and sanitation, strictly speaking
the ageing process has scarcely been challenged.
Average life expectancy is a poor indicator of the rate of ageing because
it lumps together all the many causes of death, from infant mortality to
road accidents. An alternative measure is the mortality rate doubling
time (MRDT), the time it takes for the probability of dying to double.
The beauty of this measure is that it eliminates the effects of accidental
death,such as fatal infections, predation or being run over by a bus. It
only measures the rate of physical decay associated with ageing. Fruit flies
have an MRDT of about 10 days, mice 3 months, and humans 8 years.
Caleb Finch, a neurobiologist at the University of Southern California, Los
Angeles, has found the same 8-year mortality-doubling period in American
women in 1980, Australian civilians during the Second World War and Australians
in Japanese prisoner-of-war camps in Java.
Steven Austad of the University of Idaho has even estimated that people living
in the Stone Age, despite being 150 times as likely to die in any given year,
had the same rate of ageing as we do today.
So what exactly does ageing do to the body? Needless to say, not all the
changes are life-threatening. Starting at the surface, cells in the outer
layer of skin-the epidermis-die faster than they can be replaced with
fresh cells coming up from below (See Inside Science No. 78). This leads
to thinning and wrinkle formation (Figure
1). Furthermore, these new cells become increasingly disorganised. In
the layer just below the dermis -strength is supplied by collagen
fibres. But with increasing age, the formation of crosslinks between these
molecules renders them less and less flexible (see Inside Science No.110).
Over the years, there is a stiffening of the skin's elastin, the protein
that gives the skin its flexibility. Sebaceous and sweat glands become less
active, making the skin more vulnerable to drying out and overheating. In
the fatty layer beneath the dermis-the hypodermis-the total number of fat
cells declines, but they accumulate in particular areas resulting in bags
under the eyes, enlarged ear lobes and a double chin. Elsewhere on the face,blood
vessels and bones are increasingly visible as a result of the overall loss
of fatty tissue. The skin becomes paler because there are fewer capillaries
near the surface, and pigment cells enlarge and gather, creating age spots.
Thanks to the constant work of bone-building cells called osteoblasts,
and bone-destroying cells called osteoclasts, our entire skeletons
are replaced every 7 years or so. As we age, the balance between bone formation
and resorption is upset, leading to a loss over a lifetime of about 15 per
cent of total skeletal mass in men, and 30 per cent in women. The loss is
particularly dramatic in post-menopausal women. In both men and women, bones
become more prone to fracture as a result of mineral depletion and increased
porosity. The flexibility of joints starts to decline from about age 20,
and by old age mobility can be severely restricted by arthritis. This is
caused by the destruction of joint cartilage, and the pain and inflammation
that ensues.
1: Genes - the good the bad and the
belated
|
The progressive decline in mental and
physical abilities caused by Alzheimer's disease is a prime example
of harmful "ageing"genes slipping through the net of natural selection. A
protein called ApoE helps to remove cholesterol from the blood, and
the gene that codes for it comes in three varieties,
e2, e3 and
e4. People who carry the
e4 gene are more prone to high cholesterol levels,
heart disease and Alzheimer's, but e2 seems to
protect against these diseases. The e2 gene is
somewhere in between. Research suggests that people who carry
e4 and also suffer from hyperglycaemia
(high blood sugar levels) in middle age experience greater declines in mental
function than those who have hyperglycaemia or e4
alone. It looks as though individuals who carry the
e4 gene are less able to repair nerve cells damaged
by high blood sugar levels.
In all populations studied so far, the e2 gene
is the most common, followed by the harmful e4,
with beneficial e2 bringing up the rear. How can
this be? Isn't evolution meant to weed out damaging
genes? To understand why is to understand why ageing is inevitable: Alzheimer's
and heart disease only strike late in life and so are likely to have only
a limited effect on reproductive fitness. |
Use it or lose it
Loss of strength
EXERCISE can help elderly people to maintain bone density, and the same is
true of muscle strength. "Use it or lose it" seems to be the rule, with neglected
muscle cells being turned into connective tissue and fat. But no matter how
much you exercise, there is an inevitable, slow decline in strength. This
is due to a poorer blood supply to muscles and less effective nervous
stimulation. Mitochondria, the powerhouses of cells, may also become
less efficient in muscle cells.
The ability of the heart to pump blood around the body declines as a result
of thickening of the wall of the left ventricle. Meanwhile, the smooth muscle
layer surrounding blood vessels becomes thicker and stiffer due to a buildup
of calcium and collagen,making the vessels less able to transmit pressure
waves from the heart. In atherosclerosis, arteries may become clogged by
buildup of fatty deposits on their inner lining.
Intelligence, at least as measured by IQ, peaks between the ages of 18 and
25, then slowly declines. Both short- term and long-term memory deteriorate.
The loss of long-term memory seems to be a problem of retrieval rather than
storage-the memories are in there somewhere, we just don't know where we
filed them. Our brains shrink as we age, losing between 5 and 10 per cent
in weight between the age of 20 and 90 years. One tenth of all the brain
cells we have when we are in our 20s will be lost by the age of 65. But things
aren't nearly as bad as the statistics suggest. While we may lose a lot of
neurons, the density of synapses- the connections between nerve cells-may
actually increase, offsetting much of the loss of mental agility. Unfortunately,
some old people are afflicted by Alzheimer's disease, which causes a more
alarming loss of function (see Box 1).
Elderly people are also vulnerable to infections that their immune systems
are encountering for the first time. This is particularly true of flu viruses,
which mutate into new strains every year. This loss of primary immunity
results from a decline in the body's limited stock of "virgin" T
cells-immune cells responsible for spotting foreign molecules (antigens)
that the body has never come across before (see Inside Science Nos. 7 and
8). At the same time, elderly people are more prone to autoimmune
diseases, where the immune system attacks the body-for instance in rheumatoid
arthritis and Alzheimer's.
These are some of the outward signs of ageing, but what happens at the molecular
level? Here there is a paradox. It turns out that two of the most important
substances for life do most of the damage: oxygen and sugar. Aerobic
respiration, in which oxygen is used to break down complex organic molecules
such as fat and carbohydrate to release energy, produces highly reactive
by-products called free radicals (see
Figure 2).
These have the potential to wreak havoc, particularly in the
vicinity of mitochondria, where respiration occurs. As a result, the small
but vital amount of DNA inside mitochondria is especially vulnerable. Less
reactive radicals, such as hydrogen peroxide, diffuse through the cell and
into the nucleus, where they may damage the DNA in chromosomes as well. Fats
also come under attack wherever they occur in the body, for example in membranes
or as part of hormones and eye pigments. The harmful form of blood-borne
cholesterol, low-density lipoprotein (LDL) is also attacked-which
might seem a good thing. But when LDL is oxidised by free radicals, it changes
into a form which cannot be recognised as "self" by the immune system, making
it a target for autoimmune attack. This process may contribute to the development
of fatty plaques in arteries (see Figure 3).
Fortunately, antioxidant vitamins such as E and C can soak up free radicals.
Enzymes also play a part. Catalase, for example, converts hydrogen peroxide
into water. It has been estimated that there are as many as 10 000 instances
of free radical damage per cell per day. Most of these chemical dents are
patched up by the body's repair mechanisms, but not all. Over the years,
the damage accumulates.
Sugars can also harm vital molecules. Glucose binds to proteins in a process
called glycosylation. For example, the cross-links that make collagen less
flexible are the result of glycosylation. The effects of this deterioration
can be seen everywhere in the body where this long-lived protein is found,
especially in arteries, tendons, ligaments and the lungs. When collagen in
the walls of arteries is glycosylated it tends to trap passing proteins,
and this may be another factor in the accumulation of LDL cholesterol. All
proteins are prey to glycosylation, which makes them less soluble and less
likely to be broken down. There is now some evidence that glycosylation is
behind the formation of Alzheimer's plaques in the brain.
 |
Figure 3: Plaques may form in
a blood vessel when its lining is damaged. Platelets and LDL cholesterol
penetrate the endothelium. Smooth muscle and Immune cells then ingest LDL
and become foam cells |
One leading theory argues that ageing is due to the buildup over a lifetime
of unrepaired damage to DNA, lipids and proteins,particularly that caused
by free radical attack and glycosylation. This is the error accumulation
theory. Although young bodies do not begin to age until puberty, older
bodies really are like ageing cars- no matter how much the rusty bodywork
is patched up and the engine retuned, they'll never look as good or run quite
as well as they did when they were brand new. Enzymes and long-lived structural
proteins become less efficient, mainly as a result of direct damage, but
sometimes because there are unrepaired errors in the blueprint for proteins,
DNA. These mutations are passed on whenever a cell divides. The worst kinds
of mutations cause uncontrolled cell division-cancer. Mutations in tumour
suppressor genes called p16 and p53, for example, have been
found in many cancers.
Luckily for us, even after DNA repair mechanisms have failed, the body still
has a few tricks left to prevent the runaway cell division that causes cancer.
One involves the shortening of structures called telomeres at the tips of
chromosomes. These lengths of DNA do not code for proteins- they are junk
DNA-but are nevertheless essential for the successful duplication of
chromosomes during cell division The mechanics of DNA replication dictate
that a chromosome cannot be copied right to the end, so a little bit of the
expendable telomere is lost every time a cell divides. When it becomes too
short, however, genes are activated that shut down cell division. This limits
the number of divisions that each cell can undergo, and hence protects against
cancer. But in protecting itself against uncontrolled cell division, the
body also limits the capacity of tissues and organs to renew themselves.
Of course, things aren't quite that simple, because some somatic tissues
need to go on dividing however old you are. And if the telomeres in our germ
cells shortened every time they divided, our species would be destined for
extinction. An enzyme called telomerase saves them from this fate by rebuilding
the ends of chromosomes (Box 2). The gene
for telomerase is only switched on in cells that need this licence to divide
indefinitely. But if genes that suppress telomerase production in an ordinary
cell are damaged, it may develop delusions of immortality and become cancerous.
Why oh why?
Evolutionary ideas
THESE mechanisms help to explain "how" we age, but not "why". Only
evolutionary theories can do this. Natural selection favours characteristics
that ensure the survival of the optimum number of offspring to reproductive
maturity in a given environment. One might think that if an individual had
genes that allowed it to continue living and breeding for longer than its
fellows, these would automatically be favoured by natural selection. But
this would be to ignore environmental factors. In the wild, animals rarely
die of old age, because predators, disease or seasonal changes such as falling
temperatures and reduced availability of food get them first. To be successful
in evolutionary terms, they must reach sexual maturity quickly and produce
offspring before the food runs out or they are eaten, injured or fatally
weakened by parasites. It is easy to forget that until relatively recently
in our evolutionary history, this was also the lot of
Homo sapiens.
The consequence of living in a dangerous environment is that the effectiveness
of natural selection decreases with increasing age. This means that genes
that are harmful late in life are unlikely to be weeded out of the gene pool,
because the individuals who carry them will already have passed them to their
offspring. Take the gene that causes Huntington's disease. The jerky
movements,slurred speech and steadily worsening dementia that characterise
this illness only appear in middle age, by which time the gene responsible
may already have been passed to the next generation. This is even more likely
if the harmful gene has the effect of increasing reproductive capacity in
early life. This is known as antagonistic pleiotropy (a pleiotropic
gene is one that controls more than one attribute). For example, testosterone
is essential for male fertility, but it also suppresses the immune system,
accelerates the senescence of arterial walls, and is involved in the development
of prostate cancer.
 |
Figure 4: Hip replacement improves mobility in the elderly
after cartilage in the joint has worn away |
Balancing act
Saving on repairs
ANOTHER theory sees ageing as a consequence of the balancing act between
the need to repair damage and the need to minimise the resources spent servicing
DNA and proteins-resources that could otherwise
be used for growth and reproduction. The disposable soma theory, proposed
by Tom Kirkwood of the University of
Manchester, suggests that while there are genes that slow or even prevent
ageing, their price may be impaired reproductive success in early life. Kirkwood
believes that energy for growth and reproduction is made available by reducing
the accuracy with which somatic cells are copied. Immortal germ cells,on
the other hand, are copied, repaired and screened with a very high degree
of accuracy. They are like generals living in luxury far from the heat of
battle, while their expendable troops slug it out in the front line.
ary theories suggest that ageing is inevitable, despite our
bodies' capacity for self-repair. They seem to say that no matter how much
people exercise or pop vitamin pills, this will not add a single day to their
maximum life span. This pessimistic conclusion seems to be borne out by animal
experiments. Exercise, for example, increases the average life span of rats
in a minor way, but it has no effect on maximum age. Physical exertion in
people improves fitness, muscle and bone strength, and decreases body fat,
countering diseases of middle age and improving quality of life, but it does
not affect later life ailments. The story is the same for antioxidant vitamins
such as A and C, which have little or no effect on ageing and may be harmful
in excess. Fruit and vegetables seem to improve average life span, which
is welcome, but there is no evidence that eating your greens will help you
beat Madame Calment's longevity record.
Only one sure-fire way has been found to delay ageing all over the body-food
restriction. Rats that eat between 60 and 70 per cent fewer calories than
those allowed to eat as much as they like remain energetic for longer and
have stronger immune systems and better memory. Their tissues suffer less
oxidative damage, and their tendons and ligaments stiffen more gradually.
They not only live to a greater age on average, but the longest-lived rats
on low-calorie diets may survive up to 40 per cent longer than well-fed animals.
A clear verdict has yet to emerge from primate and human studies, but for
the moment this looks like the best bet for combating ageing. It should be
remembered, of course, that the rat experiments were carried out under highly
controlled conditions, and for people the dangers of malnutrition may far
outweigh any possible benefit.
Magical "elixirs of life" have been sought for centuries, and
science has only just joined in the quest. But there is cause for optimism.
As Kirkwood points out, just because ageing is inevitable from an evolutionary
standpoint doesn't mean life span cannot be modified. In fact, he says, his
disposable soma theory suggests the opposite. If ageing is due to the
accumulation of faults in cells as a result of an evolved tendency to economise
on maintenance and repair, then treatments that reduce cells' exposure to
this damage or enhance cell maintenance could actually begin to reverse ageing.
The idea of an elixir of life may not be so daft after all.
2: Engineered for a longer life
|
IN A few years' time, the Human Genome
Project will finish decoding the blueprint for human life. But it will
be some time before the secret of delaying ageing is unlocked, because senescence
is controlled by a plethora of genes, and unravelling their effects will
take many more years.
Animal studies have already indicated where to start in discovering the genetic
basis for ageing. In August last year a gene was found in the roundworm
Caenorhabiditis elegans that, if faulty, leads to rapid ageing in
the presence of high oxygen levels. The gene, mev-1, codes for part
of an enzyme that tackles a free radical called superoxide, which
accelerates ageing. In November, a gene was discovered in fruit flies
(Drosophila melanogaster) that, in a mutant form, allows the fly to
live 35 per cent longer than average. They dubbed it Methuselah. It
also helps the flies to shrug off stresses such as starvation, heat and the
poison Paraquat,which generates free radicals. Both discoveries lend support
to the disposable soma theory, which argues that ageing is a result of organisms
economising on cell repair and maintenance in order to pour more resources
into development and reproduction.
There was much excitement in January last year when scientists announced
that they had given cultured human cells the ability to rebuild their telomeres.
These chromosome structures are thought by some to be the cell's "ageing
clock", because they are shortened every time the cell divides until further
division becomes impossible. When the ageing cells were given an active gene
for telomerase -the enzyme that restores telomeres-they were rejuvenated
and began to divide vigorously. The cells had apparently "turned back the
clock". But before you buy shares in Telomere Biotech, bear in mind that
brain cells age, even though they never divide and so never lose their telomeres.
Using telomerase to encourage cells to divide rapidly raises the spectre
of uncontrolled cell division - cancer. |
FOREVER YOUNG What determines how long we live? It may all
go back to how tough our stem cells are. A team at the University of Kentucky
has evidence that supports the theory that we age because our stem cells
essentially run out of juice and can no longer cope with the degree of cell
turnover needed to keep our organs young. Sylvia Pagán Wesphal
investigates research that raises the possibility of extending life by altering
or replacing our stem cells to make them better at withstanding DNA damage.
http://www.newscientist.com/exc/enews.jsp?id=ns99992556
|
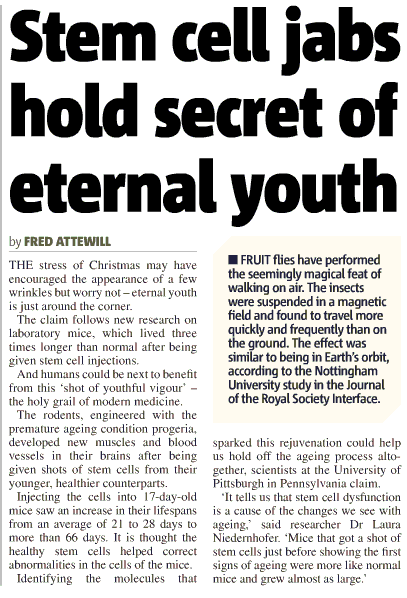 |
|
|
|
|
|
|
|
|
|